Eclogite: Diamond-Encrusted Ancient Seafloor
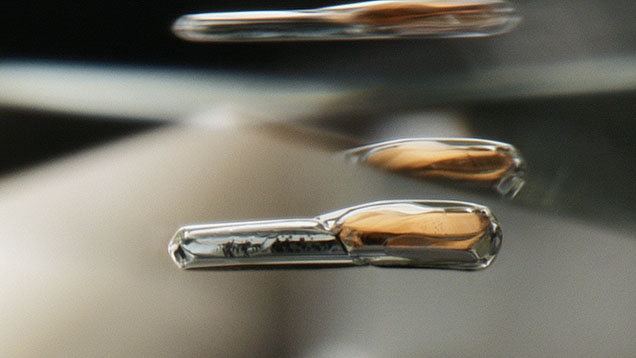
THE LINK BETWEEN ECLOGITE AND DIAMONDS
Earth’s surface is divided into tectonic plates, and the ones that make up the ocean floor are destined to sink. Sheets of oceanic crust typically bend and sink down into Earth’s interior at so-called subduction zones. Occasionally these banished slivers of ancient seafloor may become a sort of unexpected canvas to be painted with a sparkling sea of diamonds. In fact, ancient seafloor rocks that have been incorporated into old and thick parts of continents are one of the most important mantle substrates or “host rocks” for diamond growth (Stachel et al., 2022a). This particular host rock, known as eclogite, can be found as distinct fragments, called xenoliths, at some diamond mines and is also represented by mineral inclusions in diamonds (figure 1).
Our current understanding of where and how diamonds crystallize is largely based on mineral inclusions trapped inside them. The diamonds we mine were brought up to Earth’s surface by kimberlites and related magmatic rocks, but they did not crystallize directly from this magma. Rather, the diamonds formed by independent processes and are usually millions or billions of years older than the kimberlitic magmas that inadvertently swept them up to the surface. Most diamonds (~98%) were formed at a depth of about 150–200 km within the continental lithosphere, essentially in old and thick parts of continents. Based on the relative abundance of mineral inclusions in a studied set of 2,844 diamonds, the three principal mantle host rocks here are peridotite (65%), eclogite (33%), and websterite (2%) (Stachel and Harris, 2008). Eclogitic diamonds constitute a significant portion of the gem diamond market, and their features embody the dynamic processes unique to Earth.
WHAT IS ECLOGITE?
Typically, eclogite refers to a colorful red and green metamorphic rock that is made up of two key minerals: grossular-almandine-pyrope garnet and omphacitic clinopyroxene (figure 2) (Winter, 2010). Additional minerals sometimes present include rutile, coesite, kyanite, and iron-rich sulfides. All of these can be encountered as mineral inclusions in diamond. Eclogite can form in several ways, but the dominant process involves oceanic crust sinking down into the mantle (by subduction). The basalt and gabbro that make up oceanic crust undergo a transformation, known as metamorphism, as a result of changes in pressure and temperature that cause some minerals to break down and new ones to form.
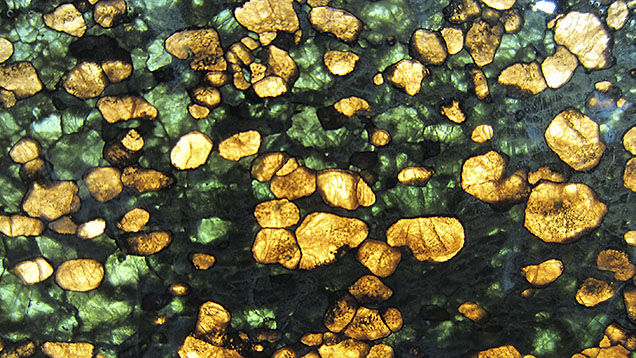
Metamorphic rocks are sometimes classified into groups, called metamorphic facies, based on the pressure and temperature conditions at which they formed. One of these groups is known as eclogite facies. Any given metamorphic facies encompasses metamorphic rocks that form under the same range of pressure and temperature conditions, regardless of what the exact starting rock, or protolith, might have been. Figure 3 shows where the eclogite facies and other metamorphic facies fall within a pressure vs. temperature diagram. When basalt or gabbro from the oceanic crust get subducted, they metamorphose through zeolite and blueschist facies before transforming into eclogite at depths of about 50 km.
If the eclogite were carried deeper still, the clinopyroxene would begin to break down between 300 and 400 km, followed by the appearance of a new phase, stishovite (SiO2) (Irifune and Ringwood, 1987). At this point, it would technically not be eclogite but could be described by the more general terms metabasalt or metagabbro. With varying pressure and temperature, the mineralogy can change drastically, even if the bulk composition of the rock remains unchanged.
ANCIENT SEAFLOOR AND THE PROCESS OF SUBDUCTION
Inclusions (figure 1) and xenoliths (figure 2) show that eclogite has served as a host rock for diamond growth at depths of about 150–200 km. But that eclogite has actually traveled a long way from its birthplace. Those same eclogitic inclusions and xenoliths have chemical and isotopic characteristics indicating that the protolith originated at Earth’s surface as oceanic crust. Present-day ocean floors are composed of oceanic crust with an underlying layer of mantle peridotite, which together form a rigid plate called lithosphere that “floats” upon the convecting upper mantle because it is less dense (figure 4). Oceanic crust is generated at mid-ocean ridges, where the two plates on either side of the ridge spread apart from one another. The gap left between the diverging plates is filled by rock that flows up from beneath and partially melts, producing the magma that will make up newly formed crust. When these magmas erupt at the seafloor, they cool quickly and form a dark, fine-grained rock called basalt. At deeper levels within a mid-ocean ridge, the magma cools slowly and crystallizes as coarser-grained rocks, chiefly gabbro.
Oceanic crust is produced continuously at mid-ocean ridges on the ocean floor. Old crust is pushed farther from the ridge as new crust is produced. Old oceanic crust will cool, increasing its density. After millions of years, and driven by continual mid-ocean spreading, the oceanic crust will eventually collide with continental crust, which is generally much less dense due to its different mineralogy and elemental composition. When the oceanic crust collides with continental crust, the large difference in density leads the oceanic crust to subduct into the upper mantle beneath the continental crust (figure 4).
Subduction is the process by which a plate on the earth’s surface moves beneath another and sinks into the mantle. During subduction, oceanic crust undergoes a progressive sequence of metamorphic reactions as pressure and temperature increase with depth (figure 3). This increases the density of the rock, which may continue to subduct until it transforms into eclogite. Occasionally, subducted eclogite is incorporated into the deep roots beneath continents, where the majority of diamond formation occurs, as shown by the green and orange lenses in figure 4.
Geoscientists can inspect aspects of eclogite geochemistry and isotopic composition to reconstruct its history. For example, when basalt forms at a mid-ocean ridge, it has an oxygen isotope composition that is similar to the upper mantle source region from which it was derived. When seawater interacts with oceanic crust, however, it can change the oxygen isotope composition of the rock, shifting it away from the original mantle-like value. Oxygen isotope compositions of minerals in eclogite that are outside the range of the typical ambient mantle are considered evidence that the eclogite had a protolith that was exposed to seawater prior to subduction (Jacob, 2004; Korolev et al., 2018, and references therein).
Eclogites also have relatively high concentrations of sodium compared to many other mantle rocks. This sodium comes from plagioclase in the oceanic protoliths, but during subduction the plagioclase becomes unstable and breaks down, with its constituent elements—including sodium—redistributed to new minerals formed during metamorphism.
With the proper analytical equipment, elements with extremely low abundance (e.g., concentrations at the parts per million or billion level) can be measured in eclogite minerals. Among these are the rare earth elements (REE), which are a group of 17 elements including the lanthanides (lanthanum, cerium, praseodymium, neodymium, promethium, samarium, europium, gadolinium, terbium, dysprosium, holmium, erbium, thulium, ytterbium, and lutetium) plus scandium and yttrium. Slight differences in the behavior of these elements during geological processes can lead to changes in their relative concentrations, imparting a sort of chemical imprint of a rock’s history. REE concentrations in igneous rocks can be inherited from their magmatic sources and subsequently modified by melting and other processes in the earth.
In many mantle rocks, REE patterns will have a smooth trend, with the concentration of europium being intermediate to the elements samarium and gadolinium (figure 5). Some eclogites, however, may have a positive or negative europium “anomaly” when compared to samarium and gadolinium (figure 5). The mineral plagioclase tends to have strong positive europium anomalies, as europium incorporates into plagioclase very strongly relative to other REE. Consequently, an igneous rock that accumulates plagioclase (such as gabbro in oceanic crust) may inherit a positive europium anomaly. A rock that forms from a magma that previously crystallized plagioclase (such as an oceanic lava) will have a negative europium anomaly. As plagioclase is only stable in the shallow portions of the earth (<30 km, approximately), eclogites with detectable europium anomalies are generally inferred to have protoliths that formed at shallow depths. Seeing this signature in mantle-derived rocks, such as eclogite xenoliths at a diamond mine, requires that those rocks formed near the surface and were carried down into the mantle by subduction (and in the case of xenoliths, these rocks have been brought back up again by kimberlites). Eclogite xenoliths really have come a long way.
RECYCLED CARBON AND ITS CONTRIBUTION TO DIAMOND GROWTH
Not all carbon atoms are identical. By paying close attention to differences in their mass (carbon isotopes), geologists have observed that diamonds formed in eclogite are distinguished by an unusual signature (figure 6). The signature suggests that eclogitic diamonds contain “recycled” carbon that was subducted from Earth’s surface down into the mantle, where diamond growth occurred (figure 4).
A typical carbon atom has 6 protons and 6 neutrons in its nucleus, which sum to give its atomic mass, 12. Carbon-12 (12C) is the most common isotope of carbon. About 1% of naturally occurring carbon atoms have an extra neutron and therefore a mass of 13. Carbon-13 (13C) is the second-most abundant isotope of carbon. Given that 12C and 13C have different masses, they behave slightly differently during chemical reactions. When plants grow, for example, photosynthesis favors 12C. As a result, plants are enriched in 12C (and depleted in 13C) relative to the initial carbon dioxide in the atmosphere. Scientists can gain insight into many carbon-bearing materials by measuring the relative proportions of 12C and 13C. For any measurement, the 13C/12C ratio is reported in terms of how different it is from an agreed-upon standard (a reference known as the Vienna Pee Dee Belemnite). This deviation is written as δ13C (pronounced “delta C thirteen”) expressed in parts per thousand (per mille, ‰) and is calculated as δ13C = [(13C/12C)sample/(13C/12C)standard−1]×1000.
Figure 6 shows δ13C values for peridotitic and eclogitic diamonds. The histograms convey information about sources of carbon and potentially about the chemical process of diamond formation as well. In both histograms, the large peak centered at –5‰ corresponds to the average composition of carbon spread throughout the mantle (Cartigny, 2005). Eclogitic diamonds, however, possess a conspicuous tail of negative values or “light” isotopic compositions. These negative values are one of the most debated features of diamond geology, with no firm consensus on the exact processes at play (Cartigny, 2005).
The most accepted general view is that the negative isotopic trend of eclogitic diamonds stems from subducted carbon (Li et al., 2019; Stachel et al., 2022b). Subducted carbon is thought to mix with the ambient carbon already present in the mantle to give the range of observed carbon isotopic characteristics in eclogitic diamonds (figure 6). The recycled carbon could come in the form of sediments and altered oceanic crust containing organic matter and carbonates (biogenic and abiogenic) (Li et al., 2019). Taking into account the covariations in nitrogen concentration and isotopes suggests that eclogitic diamond formation involves multiple subducted ingredients that mix in complex ways during the process (Stachel et al., 2022b). Although there are unresolved details, the evidence is strong that a portion of the carbon in eclogitic diamonds is subducted. These diamonds are a physical manifestation of the deep and ancient geological carbon cycle.
ECLOGITIC DIAMOND AGES
Through the incredible phenomenon of radioactive decay, many rocks and minerals have a sort of built-in natural clock. Radioactive decay is the process whereby unstable radioactive elements break down over time, with different elements each having a predictable decay rate. Several decay systems apply to various types of inclusions in diamonds, and their analysis has led to our understanding that diamonds are millions to billions of years old (Smit and Shirey, 2019). The oldest diamonds are 3.5 billion years old, and diamonds have been forming episodically since that time, spanning three-quarters of Earth’s history. Their antiquity combined with their deep mantle origin makes diamonds a unique record of large-scale geological evolution.
For example, diamond ages may provide a time stamp to mark the beginning of modern-style plate tectonics. Many of us are at least vaguely familiar with the notion that roughly 200 million years ago, the continents to the east and west of the Atlantic Ocean were nestled together in the supercontinent Pangaea. The Atlantic grew and opened up as Pangaea broke apart. The subduction zone and mid-ocean ridge spreading center depicted in figure 4 are integral mechanisms that explain how tectonic plates move, how new oceans form, and how old ones close and vanish. This dynamic surface structure is unique to Earth. Our planet did not always have plate tectonics, however, and at one point, its surface was a giant magma ocean. So when exactly did things change and the processes we observe today take hold?
Geologists have used several methods to constrain the timing of when plate tectonics began, including clues from diamonds. Comparing samples of different ages reveals that they are not all equal across time. Diamonds older than 3.0 billion years are exclusively peridotitic, whereas eclogitic diamonds only become prevalent among those younger than 3.0 billion years. This change arguably marked the first major episode of subduction of oceanic crust beneath a continent and the onset of the Wilson-cycle style of movements that characterize modern plate tectonics (Shirey and Richardson, 2011). Effectively, this model suggests there are no eclogitic diamonds older than 3.0 billion years because oceanic crust was not yet being subducted and incorporated as eclogite into the continental lithospheric mantle.
ECONOMIC IMPORTANCE OF ECLOGITIC DIAMONDS
Finding an economic diamond deposit is no trivial matter. Even after locating one or more kimberlites (figure 7) or lamproites, it is a challenging exercise to evaluate the quantity and quality of diamonds. A key variable that can make or break a potential mine is the presence of eclogitic diamonds. In some deposits, eclogitic diamonds are much more abundant than might be expected based on the relatively small amount of eclogite in the mantle.
Most of the continental lithospheric mantle is made up of peridotite (>95 vol.%; figure 4). Conversely, eclogite has a low global abundance (<5 vol.%) (Dawson and Stephens, 1975; Schulze, 1989), yet some mines can contain much higher abundances of eclogitic diamonds, to the point where they dominate the diamond population, such as at Koidu (Sierra Leone) and Orapa (Botswana). One in three diamonds have eclogitic vs. peridotitic mineral inclusions, indicating a relatively large proportion of diamonds are associated with eclogite (Stachel and Harris, 2008). While not all eclogites are associated with diamond, small volumes of eclogite in some kimberlites could be indicative of diamond-rich deposits.
Kimberlites can be very small (as small as 50 m across) and show very little topographic relief, making them difficult to find. Over millions of years, these sites may become completely covered by vegetation or water (forming lakes). In these cases, kimberlites can be located by exploiting their physical properties, as they erupt through existing rock and may have different magnetic or gravimetric properties from the surrounding material. Aerial surveys that passively examine the landscape, searching for gravitational or magnetic anomalies, could potentially identify a kimberlite.

A more direct approach is to use kimberlite indicator minerals (figure 8). Even in kimberlites that are diamond-bearing, diamonds may be present in abundances at the parts per million level. Conversely, rocks that are associated with diamonds are generally much more abundant and easier to locate. Minerals in these rocks commonly have elemental compositions that reflect the rock from which they derived and, occasionally, an association with diamond itself. For example, there is an observed association between diamond and mantle peridotites that are very chemically depleted (i.e., experienced substantial melting in the mantle, removing elemental components from their structure) (Gurney, 1984). The compositions of the garnets in these peridotites are characterized by low calcium contents and high chromium contents (Grütter et al., 2004). Garnets in eclogite are very different and instead have low chromium contents and variable calcium (Grütter et al., 2004). Given the disproportionate number of eclogitic diamonds compared to the global abundance of eclogite, even a small number of eclogitic garnets, such as the orange grains in figure 8, may indicate an economically valuable deposit. Minerals within kimberlite can be redistributed by rivers or glaciers, so if a garnet with composition similar to those associated with diamond is found in stream sediment or glacial till, this could indicate that a kimberlite deposit—possibly a diamond-bearing one—occurs upstream. When identified, a kimberlite deposit could one day be developed into an operating diamond mine.